
Science
Introduction
Although we spend nearly a third of our lives sleeping, the function of sleep remains mysterious. Throughout the animal kingdom, animals sleep for hours each day, despite it leaving them defenseless and unable to feed or mate. The evolutionary pressure to maintain sleep has resulted in some unusual evolutionary adapations, such as the unihemispheric slow wave sleep seen in certain marine mammals, where basically half of their brain is asleep at a time, so that they can continue to move and surface to breathe.
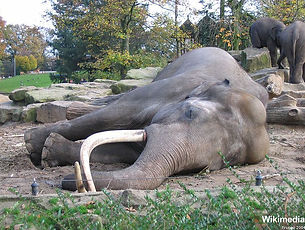
In addition, the role of sleep in human health and disease is being increasingly recognized. Sleep deprivation can cause behavioral, cognitive, and metabolic impairment in humans. Further, sleep disorders such as sleep apnea, narcolepsy, restless legs syndrome, and insomnia are common and significant causes of human morbidity. Despite the importance of sleep, the underlying molecular mechanisms remain poorly understood. Understanding the molecular basis of sleep may provide novel targets for the diagnosis and treatment of sleep disorders.
underlying sleep homeostasis could have implications for sleep disorders involving excessive daytime sleepiness, such as idiopathic hypersomnolence. The recent discovery that the fruit fly Drosophila melanogaster sleeps allows for the use of powerful genetic techniques to identify and characterize the molecular and cellular pathways involved in sleep.
Genetic Analysis of Sleep
We have taken a forward genetic approach to identify novel sleep mutants, in order to gain insights into the molecular mechanisms underlying sleep. Using such an approach, we identified and characterized WIDE AWAKE, the first clock output molecule shown to specifically regulate the timing of sleep onset (Liu et al., Neuron 2014). WIDE AWAKE acts as a key molecular intermediary that translates
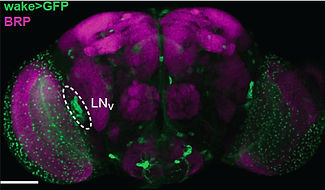
timing information from the core oscillator to regulate sleep. Loss of WIDE AWAKE leads to a decrease in sleep time and also a marked defect in the timing of sleep onset. WIDE AWAKE is expressed in arousal-promoting clock neurons (the large ventrolateral neurons), and its levels rise during the day under control from the core oscillator and peak at dusk. WIDE AWAKE upregulates the GABA-A receptor RDL in a time-dependent manner, and patch-clamp analysis from the large ventrolateral neurons of wide awake mutants reveals a significant decrease in GABA-evoked current and also a significant increase in the excitability of these neurons at night. Thus, WIDE AWAKE, under clock control, silences a key arousal circuit at dusk, promoting sleep onset at that time. WIDE AWAKE, under clock control, silences a key arousal circuit at dusk, promoting sleep onset at that time.
The focus of our research is the molecular and genetic basis of sleep. Our goal is to use the power of high-throughput forward genetics in Drosophila to identify novel genes and neural circuits that regulate sleep and then study the importance of these genes in mammals by generating knockouts in mice. To accomplish these aims, we use a multi-pronged approach, including genetic analyses in flies and mice, molecular and biochemical assays, immunocytochemistry, behavioral readouts, patch-clamp electrophysiology, and in vivo functional imaging.
There is a single homolog of WIDE AWAKE in mice and humans, and strikingly, we find that the mouse homolog is enriched in the suprachiasmatic nucleus, which is the master circadian pacemaker in mammals. Furthermore, we find that it is specifically enriched in the "core" region of the suprachiasmatic nucleus, where its expression overlaps with the GRP peptide. Interestingly, this "core" region in mammals and the large ventrolateral neurons in flies both directly receive light input into the circadian clock, and thus these data suggest that the function of WIDE AWAKE will be conserved in mammals. We are continuing to study the function of WIDE AWAKE in both flies and mice.
Neural Circuits Underlying Motivated Behaviors
All of our behaviors--our thoughts, our feelings, our actions--depend upon the computational power derived from the coordinated actions of many neurons. Dissecting the cellular basis of behavior can be challenging in mammals, whose brains have many millions to billions of neurons. In contrast, the fruit fly nervous system contains ~100,000 neurons.

Furthermore, fruit flies are capable of complex behaviors, including learning and memory, courtship, aggression, as well as sleep and circadian rhythms. Thus, Drosophila is an outstanding model for identifying and characterizing the neuronal circuits underlying behaviors, such as sleep and feeding.
Our data thus suggest an alternative model to the "somnogen" hypothesis, and argues that plasticity of a dedicated sleep homeostatic circuit encodes sleep drive, providing an explanation for the gradual buildup and dissipation of sleep drive. We are continuing to study this circuit, as it provides a unique entree for understanding the molecular and cellular basis of sleep homeostasis.

a significant increase in activity when the animal is protein starved. Interestingly, this circuit not only promotes protein feeding, but also suppresses sugar intake, and it does so via distinct terminal branches. Strikingly, this circuit undergoes branch-specific plasticity, such that after protein starvation the branch signaling to the protein feeding circuit increases its size and activity, while the branch signaling to the sugar feeding circuit remains unchanged. This branch-specific plasticity allows for the regulation of protein and hunger consumption on distinct timescales; strong protein drive leads to a temporary inhibition of a food typically preferred at baseline (sugar) and persistent desire for the food necessary for survival (protein). We have also spent substantial effort generating new genetic tools that should allow for the rapid identification and characterization of dopamine neurons regulating other behaviors.
In broad terms, sleep is regulated by circadian and homeostatic influences. In other words, the time of the day and the amount of sleep drive affect whether the organism sleeps or is awake. Work in Drosophila paved the way for the molecular dissection of the core clock mechanism, which comprises a transcriptional-translational feedback loop. However, how this core oscillator translates its timing information to regulate sleep is poorly understood. In addition, the nature of the sleep homeostat remains enigmatic, but as discussed below, we are making progress towards understanding this process. Unraveling the mechanisms
We have recently identified a critical circuit encoding homeostatic sleep drive in Drosophila (Liu et al., Cell, 2016). This circuit comprises a small subset of central complex neurons that is both necessary and sufficient for sleep homeostasis. To our knowledge, this circuit is the first described that is able to induce sleep drive when activated, even in fully rested animals. Functional imaging and patch-clamp recordings demonstrate that the activity of this circuit correlates with sleep drive in a scalable manner. Importantly, this circuit undergoes plastic changes with increased sleep need, and our data suggest that these plastic changes directly encode sleep drive. Over the past century, the dominant model underlying the homeostatic regulation of sleep drive is encoded by extracellular "somnogens." However, one long-standing issue has been that these substances are short-lived (on the order of minutes) whereas sleep drive persists for hours.
We are also studying the dopaminergic circuitry underlying behavior in Drosophila. Dopamine is a key neuromodulator in animals ranging from worms to humans, and regulates many behaviors including motor control, reward processing, learning, motivation, decision-making, and sleep/wake. Furthermore, impaired dopamine signaling plays a critical role in a wide variety of human diseases, including schizophrenia, Parkinson's disease, and addiction. Recently, we have discovered a small subset of dopamine neurons that encodes protein hunger, the first such circuit identified (Liu et al., Science, 2017). Although many people consider hunger as a binary state, it turns out that animals can have nutrient-specific hunger. The circuit we recently identified is both necessary and sufficient for protein hunger and exhibits
